Overview
Some of Darwin’s earliest supporters found evidence of evolution in fossils. Fossils continue to inform our ideas of evolution as Neil Shubin tells us. He describes his search for a fossil link between fish and land animals and the excitement of finally finding it. In a review of session 2, Sarah Tishkoff reminds us of what fossils tell us about human evolution and migration. Further evidence of evolution comes from comparing DNA sequences. Hopi Hoekstra studies the impact of genes and the environment on the evolution of specific phenotypes. She found that populations of mice living on white sand beaches were more likely to evolve light colored fur than populations in areas with dark soil. (Why do you think this happens?) In about half of the populations, this change was due to a single mutation in the MC1 receptor. In an interesting example of convergent evolution, the same mutation causes coat color variation in some dogs and may have caused coat colors to vary in woolly mammoths!
Finding Tiktaalik, the Fossil Link Between Fish and Land Animals
Concepts: Study of fossils, Tiktaalik
00:00:07.22 My name is Neil Shubin, I'm from the University of Chicago.
00:00:10.01 And I'm going to talk today about finding Tiktaalik.
00:00:12.06 My life's quest began in graduate school, in the second year
00:00:16.26 when I saw this exact slide. This is the slide my professor
00:00:19.29 showed back in 1986, and it showed what we knew at the time as
00:00:25.17 the fish to tetrapod transition, which is the transition from life in water
00:00:29.19 to life on land, as seen by these cartoons of these fossil creatures.
00:00:33.12 The one on top is a fish from 390 million years ago, the one on the bottom
00:00:38.18 is a tetrapod from about 365 million years ago. I remember as a student
00:00:43.10 seeing this diagram for the first time and thinking, "Holy cow!
00:00:46.20 What a first class scientific problem!" How did fish evolve to walk on land? It
00:00:52.00 seemed so utterly impossible go to from the thing on top to the thing
00:00:54.28 on the bottom. And that's what became my quest. So like any
00:00:58.00 good paleontologist, I applied the paleontological toolkit to try to find
00:01:01.09 an intermediate, say some sort of creature between these two.
00:01:04.14 And so what do we do when we try to find a new place to discover fossils?
00:01:08.18 What we do is we look for places in the world that have three things.
00:01:11.27 The first is rocks of the right age, you need rocks of the right age
00:01:16.08 to answer whatever question interests you. So in this case, clearly
00:01:19.19 we want something between 390 and 365 million years old.
00:01:24.05 That's of a time period known as the Late Devonian. The other thing
00:01:28.08 that was really important, of course, is to have rocks of the right type.
00:01:31.00 Not every kind of rock holds fossils. Some are super heated, some are
00:01:35.06 highly pressurized. So we needed a particular kind of rock, sedimentary
00:01:39.15 rocks, and not only that, sedimentary rocks in the right environment
00:01:43.20 to hold the kind of intermediates we're looking for. So what's the
00:01:47.14 other variable? Rocks of the right age. Rocks of the right type.
00:01:50.10 Well, it does me no good if my wonderful rocks of the right age
00:01:53.27 and the right type are buried five miles underground, right? These things
00:01:57.08 have to be exposed at the surface. So what we do is we look for places
00:02:00.28 in the world where we have naked bedrock, okay? So that's it. If you
00:02:04.10 ever want to run your own expedition someday, look for places in the world
00:02:07.18 that have rocks of the right age, rocks of the right type, and rocks that
00:02:10.09 are exposed at the surface. There's another criterion, and in this case
00:02:14.06 was accessibility. And this is where I began my first scientific expeditions.
00:02:19.06 These were accessible, because I had just begun my first academic
00:02:24.12 job at the University of Pennsylvania, here at the southeastern corner
00:02:28.00 of the state of Pennsylvania. And I dug out a geological map of
00:02:31.15 the state. And I'm showing you here what is sort of relevant for our
00:02:35.21 question. If you look at this map of Pennsylvania, what you see is
00:02:39.00 everything in purple are Devonian age rocks. So I have lots of
00:02:43.22 rocks of the right age throughout the state of Pennsylvania. But what about
00:02:47.02 that second variable, rocks of the right type? Well if you want to
00:02:50.29 think about what Pennsylvania looked like in the Devonian, get Pittsburg,
00:02:54.29 Harrisburg, Philadelphia, out of your brain. And think amazon delta.
00:03:00.14 This is a cartoon of what Pennsylvania looked like 365 million years ago.
00:03:04.24 You had highlands to the east, an inland sea to the west, and a series
00:03:09.12 of rivers draining from east to west. Now if you're a paleontologist
00:03:14.01 wanting to find fossils at the cusp of the transition from life in water
00:03:18.01 to life on land, this is perfect. Because if you have the right exposures,
00:03:22.19 you can sample ancient seaways, ancient rivers and streams, even ancient
00:03:27.15 upland deposits. Now the problem is Pennsylvania doesn't have a whole lot
00:03:31.21 of exposures. So that third variable is very tough. So my initial
00:03:36.06 expeditions, if you will, became following the Pennsylvania Department of
00:03:40.24 Transportation around as they build new roads. And if we were really lucky,
00:03:45.02 what they would do is they would be digging a new road in a place of Devonian
00:03:48.23 rock. And that's what you see here. This is a set of rocks about an hour north
00:03:53.02 of State College Pennsylvania. And this expanse extends about half a mile
00:03:57.21 long. It's a big red hill of rock -- it's called Red Hill, no surprise.
00:04:01.12 But what you see in these layers is the strata, the ancient layers
00:04:05.21 of ancient streams. And this is really perfect. Almost as soon as we
00:04:10.02 hit this site in the early '90s, we started to find fossils. The first thing
00:04:15.07 we started to find were teeth the size of railroad spikes. So big monsters, almost the size of
00:04:19.15 your thumb. Then we started to find the jaws of these creatures. Here's the
00:04:22.27 front end of one of these jaws. These jaws would be the length of your arm.
00:04:25.27 Alright, so they're really big. These big animals are probably about
00:04:29.13 16 feet long. Then we started to find all kinds of other fish, like
00:04:33.01 this little mashed thing here. This is actually a mashed fossil
00:04:36.25 of a lobed fin fish, which is related to lungfish today. So that's a head
00:04:41.14 and a body. But then my colleague, Ted Daeschler, who's been my partner
00:04:45.07 in all these expeditions for the last number of decades. Ted found
00:04:49.11 early tetrapods. One day I had left, one week I had left to do
00:04:53.28 expeditions elsewhere, and he had just discovered a series of
00:04:56.04 bones. Arm bones, leg bones, from creatures that represented
00:04:59.16 early limbed animals. And working with the National Geographic Society,
00:05:03.18 Ted and I were able to reconstruct what this ancient road in Pennsylvania
00:05:07.16 looked like 365 million years ago. I mean we had some of the first
00:05:12.10 forests, you can see this, and ancient streams, see that in the middle
00:05:16.04 here is that big monster fish, about 16 foot long fish. And then you see
00:05:20.28 a series of other kinds of fish, in particular, you see the little creatures
00:05:23.21 with limbs crawling around both on land and in the water.
00:05:27.16 Now this was great, but Ted and I realized we had a problem.
00:05:31.23 Why? We're in rocks about 365 million years old and we're already finding
00:05:38.00 pretty advanced limbed creatures. Remember what motivated this
00:05:41.02 whole quest was this whole slide I saw in graduate school.
00:05:44.22 We're not finding intermediates, we're already finding pretty advanced
00:05:47.20 limbed animals, I want something in the middle here. Well, to give you
00:05:50.18 a sense of what we're after, let's just go through some of the
00:05:52.26 traits that we look for. When you look at the creature on top, it has a
00:05:56.06 conical head with eyes on either side. The creature on the bottom
00:06:00.01 has a flat head with eyes on top. So really one of the kind of features
00:06:03.28 that changes in this transition is the shape of the head.
00:06:08.08 The other thing that changes is if you look at the creature on top,
00:06:10.27 the fish on top, you'll see the head is connected to the shoulder girdle by a series
00:06:14.21 of bones. It doesn't have a neck. Fish don't have necks where the head
00:06:18.20 can bend independently of the body. Early limbed animals, early
00:06:22.23 tetrapods, have a neck where the head can swivel independently
00:06:26.16 and it can look around as its legs are on the ground. Finally, another big feature
00:06:31.23 which obviously gives it its name, tetrapods, four-legged animal,
00:06:35.02 is fish have fins and the earliest tetrapods have limbs with fingers
00:06:39.03 and toes and wrists and ankles. We're finding a lot of fingers, toes, and wrists,
00:06:43.08 and ankles, right? We're not finding any intermediate. What Ted and I
00:06:46.14 are looking for is a flat headed fish with say fins or something in that
00:06:50.18 intermediate zone. To get that, what we had to do was move back in time,
00:06:55.02 back in time about 15 million years using analyses that other colleagues had done.
00:07:00.06 So Ted and I pulled out the paleontological rule book and began looking for
00:07:04.21 places that have rocks that are the right age, rocks that are the right type,
00:07:07.11 and rocks that are exposed. Wed had an idea to work in Brazil.
00:07:11.16 We had an idea to work in western North America. Then everything
00:07:15.28 changed one day in my office, back in the late '90s, when we had a
00:07:20.28 geological debate. And to settle the debate, I pulled out a college
00:07:24.15 geology textbook, this one right here. It's now in its eleventh edition.
00:07:28.19 So that gives you a sense of how old I am. But anyway, so we
00:07:31.01 finished the debate and I was paging through the book, and we ran
00:07:35.08 into this figure. This is the figure that changed the traction of research
00:07:39.25 for me, about 15 years ago. What it is is what we look for in a nutshell
00:07:45.01 as paleontologist. You'll see the caption, it says upper Devonian sedimentary
00:07:49.11 facies. Rocks more or less of the right age and the right type.
00:07:52.27 But here you see a map of North America, and superimposed on that
00:07:56.18 map is an interpretation of the geology of the Devonian age
00:08:00.27 rocks. Now you'll notice in the western part of North America,
00:08:03.20 there are Devonian rocks mapped as ancient oceans, but then the authors
00:08:08.05 identify three different areas, shown in red here, that had rocks
00:08:12.03 that had formed in ancient rivers and streams. Ancient rivers and stream
00:08:15.06 environments, delta systems. The first one, you've seen before.
00:08:20.01 That's the one I just showed you from Pennsylvania, that's the ongoing Catskill
00:08:23.08 project that we're working on along the roadsides of Pennsylvania.
00:08:26.29 There's another one on top here in East Greenland, that was well studied.
00:08:31.01 That was discovered in the '20s by Danes and Swedes. And
00:08:34.29 had produced a number of early tetrapods or limbed animals, in fact
00:08:38.03 the cartoon I've been showing you is the cartoon derived from
00:08:40.29 one of the fossils from this area. You see where I'm going?
00:08:43.25 Extending 1500 kilometers east to west across the Canadian arctic,
00:08:48.04 was mapped Devonian aged rock, completely unexplored. Not only that,
00:08:53.05 that rock was perfect. It was mapped as being from ancient rivers
00:08:56.03 and streams, ancient delta systems. So Ted and I were really excited,
00:08:59.13 and just a little anecdote here is, we were so excited. It's all happening
00:09:02.18 one morning, so we ran to a Chinese food restaurant across the street
00:09:05.23 and had a fortune cookie. And that fortune cookie said, "Soon you'll
00:09:09.24 be at the top of the world." So yes, here we go! We had a fortune cookie,
00:09:13.02 off we go! So anyway, this is a colorized version of one of the scientific papers
00:09:17.15 and it shows the stratigraphic section right here, and as well as the
00:09:21.07 map. And you can see, super-imposed over the map of the Canadian
00:09:25.15 arctic is a huge area, shown in red here, of Devonian age rock
00:09:30.18 So you can see it's all over. This is 1500 kilometers of section.
00:09:35.23 So now we had a new problem, instead of driving in our station
00:09:39.23 wagons to central Pennsylvania, we're now working several hundred
00:09:42.28 miles from the North Pole, where the arrow is in this slide.
00:09:46.02 To give you a sense, the nearest town is about 300 miles away.
00:09:50.06 I'm going to show you a picture of that town, with about 170 permanent residents.
00:09:54.07 This is a picture of that town in spring, Beresford, Canada. This is a big city!
00:09:58.22 And so this is resupply. Anyway, as you can imagine, much of our
00:10:02.16 supply is based on planes and helicopters. And so we're highly
00:10:06.20 dependent on aircraft. Now the way this affects science, is that the supply chain
00:10:12.23 is very long. And fossils are rocks, they're very heavy. So that means we
00:10:16.29 can't take home everything we find. So much of what we do in the field
00:10:20.26 is trying to determine whether we have really good material that is
00:10:23.20 worth spending the money to freight it home. So we started in 1998,
00:10:30.27 we had that fortune cookie in 1998. Our first expedition was in 1999.
00:10:35.02 It took us about a year to get the permits, the money, all that good stuff.
00:10:37.22 And we started with great hope in the western part of the Canadian arctic.
00:10:41.06 And you can see what that area looks like. It looks kind of flat, doesn't it?
00:10:45.15 And you can see our camp just below me here. This is Devonian rock.
00:10:49.02 So we'd wake up in the morning and essentially walk all over these
00:10:51.25 Devonian rocks, looking for the bones that are weathering on the surface.
00:10:56.02 We realized we had a big problem, this is the wrong part of the Arctic.
00:10:58.28 We were in all these rocks, which were marine rocks formed in ancient
00:11:02.22 oceans. It was pretty clear based on other people's discoveries
00:11:06.12 that we needed to hit rocks in rivers and streams. So what that meant
00:11:11.07 is using the geological map, this is a version of what I showed you
00:11:14.29 before for Pennsylvania, it applied also to the arctic. We were in the
00:11:18.24 ancient seaways, shown in blue. We had to move upstream. To do
00:11:22.12 that geologically, it meant moving east. So we retooled, the next year we went back
00:11:27.23 and we moved east, here to Southern Ellesmere Island.
00:11:30.25 And as soon as we did that, you can see these beautiful landscapes
00:11:33.29 here, of mountains and areas. And these are all red beds that contain
00:11:38.09 Devonian rocks formed in ancient rivers and streams.
00:11:41.15 Took another few years, we eventually honed in on an individual
00:11:45.17 valley that had good fossil preservation. And this is that valley here.
00:11:49.04 What you see, in front of us, is a big hole in the middle of this
00:11:52.21 slide, that shows a layer that was preserving hundreds of fossil
00:11:56.13 fish, preserved one on top of the other. So, the arctic's a big place. Fossils
00:12:01.16 -- you know they're not very big, so you're trying to find the little
00:12:03.19 tiny patches of rock that will have those fossils inside. And what we use
00:12:08.01 are the tools of geology and sedimentology. Well, we worked this layer,
00:12:12.02 and everything changed one day in 2004, when my colleague,
00:12:16.09 Steve Gates, he's shown in blue here to the left, removed a rock
00:12:19.18 from this layer. And he saw a little notch, a little v, you can see that v
00:12:24.01 in the middle of this slide. If you look very carefully you can see it.
00:12:27.11 He says, hey guys, what's this? We run over to it and we saw what
00:12:31.04 we spent multiple years looking for and several decades of a quest. What we had
00:12:35.09 looking at us here is the snout of a fish, and not just any fish, the snout
00:12:39.22 of a flat headed fish. Remember I told you the conical head and the flathead
00:12:43.05 was the big piece of this transition? What I had here, in the middle of this
00:12:46.15 slide, was a flat headed fish sticking right out at me. With any luck at all,
00:12:50.11 the rest of the fish would be preserved in the rock. So we removed
00:12:53.29 this thing and they come home at the base of the helicopter, so this is a
00:12:57.08 first year graduate student for scale. And it comes back several hundred
00:13:00.17 miles to a supply base. And as we remove this first specimen, we
00:13:07.01 found several more. So at the end of the 2004 season, we had multiple
00:13:10.08 specimens of this flat headed fish. We come back to the lab, we're really
00:13:15.27 excited. We come back to the lab and the preparers start removing
00:13:19.05 the rock, grain by grain, to expose the fossils within. This is what
00:13:23.25 Steve's specimen looked like several months after preparation.
00:13:27.14 You can see what's emerging is sort of a flat head with eyes on top.
00:13:30.15 You can see the tool that's used here to remove the specimen, after
00:13:34.29 several more months, what's exposed. Look here we sort of have a flatheaded
00:13:38.14 animal with eyes on top, even a shoulder that looks separated from
00:13:42.00 the rest of the head. Remember what began this quest? In graduate
00:13:45.23 school I saw this slide, fish on top, tetrapod on bottom.
00:13:48.25 We wanted a flat headed fish. After several decades, using
00:13:52.05 tools of evolutionary biology, using tools of centrography, using the tools
00:13:55.22 of geology, this is the flat headed fish. This is Steve's specimen
00:13:59.19 as removed from that hole. Flathead, eyes on top, has a neck,
00:14:03.14 you can see the head is separated from the shoulder, it has fins,
00:14:06.22 it has fin rays, and it has scales on its back. Like a fish, it has
00:14:10.21 scales and fins, like an early limbed animal, it has eyes on top,
00:14:15.18 it has a neck. And indeed, when we crack open the fin, what
00:14:18.06 do we find? Bones that correspond with the upper arm, forearm, and even parts
00:14:22.13 of a wrist. This is a real mix of characteristics. Found at the right
00:14:25.28 part of the fossil record. So this creature we call Tiktaalik, Tiktaalik
00:14:30.05 roseae. And you can see here, what's sort of salient about it. It has a
00:14:33.16 mix of tetrapod and fish characteristics, like a lobed fin fish it has fins,
00:14:37.26 scales, and primitive jaws. Like an early tetrapod, it has a neck, a wrist,
00:14:43.16 a flathead, and so forth. So here's a creature with lungs and gills, here's a
00:14:49.26 creature with limbs that have limb bones in them but also fin bones,
00:14:52.25 as well. Fined rays. It's really a mix between fish and tetrapod.
00:14:56.19 And when we put it in the phylogenetic analysis, the evolutionary
00:14:59.29 tree, you can see Tiktaalik drawn right in the middle here. It really
00:15:04.03 shows a fine sequence of the emergence of tetrapod characteristics
00:15:08.11 throughout the Devonian. So what's the take home message here?
00:15:12.15 The take home message here is that we can predict likely and unlikely
00:15:16.04 places to find fossils. We can, in doing that, learn about the great
00:15:20.27 transitions in the history of life. In particular, this one, the transition
00:15:23.28 from life in water to life on land. But the story doesn't stop there, it begins there.
00:15:29.10 Why? Because we can trace the bones in a creature like Tiktaalik.
00:15:33.09 LIke the arm bone, the upper arm bone, the humerus of Tiktaalik,
00:15:36.17 we can trace it all the way to other mammals and to people.
00:15:39.23 This story of the origin of the neck, the origin of a wrist, is not
00:15:44.27 just some esoteric event in the history of life. It's an event
00:15:48.19 embedded in our own bodies. The origin of the neck, the first time we see
00:15:52.09 in Tiktaalik and its evolutionary cousins, is something that is to become
00:15:55.09 our own neck. Likewise with the arm bones. So every time you
00:15:58.11 shake your head, every time you bend your wrists, you can say
00:16:01.07 "Thank you, Tiktaalik and its evolutionary cousins that lived in the
00:16:04.22 Devonian streams 375 million years ago."
African Genomics: Human Evolution
Concepts: Gene pool, allele frequency, genetic drift, neutral evolution, genetic bottleneck, founder effect, effective population size
Note: Please watch original video from time 00:00 to 6:50.
00:00:07.10 Hi, I'm Sarah Tishkoff.
00:00:08.23 I'm a professor at the University of Pennsylvania
00:00:11.02 in the Departments of Biology and Genetics,
00:00:13.24 and today I'm gonna tell you about my research
00:00:15.18 on African integrative genomics,
00:00:17.29 and implications for human origins and disease.
00:00:21.17 So in Part 1, I'm gonna tell you a bit about
00:00:23.24 human evolutionary history,
00:00:25.24 and what the implications are of that
00:00:27.20 on the patterns of genomic variation
00:00:29.18 that we see in populations today.
00:00:34.05 So I want to start by talking about some of the
00:00:35.26 key challenges in human genomics research.
00:00:38.19 And the first one is to characterize
00:00:40.27 the immense array of genomic and phenotypic diversity
00:00:44.29 across ethnically diverse human populations.
00:00:48.14 Secondly, to understand what the evolutionary processes are
00:00:51.16 that are generating and maintaining that variation.
00:00:54.14 And third, to better understand how
00:00:56.04 gene-gene, gene-protein, and gene-environment interactions
00:00:58.28 contribute to phenotypic variability.
00:01:01.27 So first let's start with the evolutionary history
00:01:05.00 of the hominin lineage
00:01:06.26 that's leading to modern humans,
00:01:10.13 which begins around the time that we
00:01:12.03 diverged from our closest genetic relative
00:01:14.04 the Chimpanzee,
00:01:15.18 sometime between 5-7 million years ago.
00:01:18.14 So shown here are some of the fossils
00:01:20.07 from the different species
00:01:22.17 preceding anatomically modern humans.
00:01:25.16 In blue are shown fossils from the oldest lineages,
00:01:30.06 and in fact one of the oldest is Sahelanthropus,
00:01:34.10 which has been dated to at least 7 million years ago,
00:01:37.29 and there's some debate about whether it even
00:01:39.14 belongs on the hominid lineage
00:01:41.09 or if it actually preceded the Chimpanzee and human divergence.
00:01:45.26 After that, in green,
00:01:47.14 we see the Australopithecus genus.
00:01:50.14 In yellow, we see Paranthropus genus.
00:01:54.09 In orange, we have the genus Homo
00:01:56.24 and the species proceeding anatomically modern humans
00:02:01.13 is Homo erectus, dated to about 2 million years ago.
00:02:06.14 And then we have the origins of
00:02:08.15 Homo neanderthalensis
00:02:11.02 and of anatomically modern humans.
00:02:13.24 Neanderthals are thought to have originated
00:02:16.00 somewhere between 300,000-400,000 years ago,
00:02:19.12 and modern humans originated
00:02:20.27 approximately 200,000 years ago.
00:02:24.03 Here's one of the best examples
00:02:26.11 of Australopithecus afarensis.
00:02:29.07 This was a set of fossils that was
00:02:31.24 discovered in the 1970's by Johanson and Gray,
00:02:36.02 named Lucy,
00:02:38.00 and Lucy was about...
00:02:41.04 she lived about 3.2 million years ago.
00:02:43.29 She was very small, only about 3 feet tall,
00:02:46.13 she had a very small brain,
00:02:48.07 and she was bipedal.
00:02:49.27 And being bipedal, in fact,
00:02:51.07 is one of the characteristics of the hominin lineage.
00:02:57.12 And, interestingly,
00:02:59.17 there have been some fossilized footprints
00:03:01.21 identified in Tanzania,
00:03:03.24 and we can see from these that there
00:03:06.08 appears to have been a mother,
00:03:08.27 from the species Australopithecus afarensis,
00:03:12.08 and she was holding the hands of her child.
00:03:14.29 And they must have been walking
00:03:16.15 in ash from recent volcanic activity,
00:03:20.06 and then that ash hardened and preserved these footprints
00:03:23.06 so that we can see them today,
00:03:24.21 and we can clearly see that they were bipedal.
00:03:29.08 So the species preceding modern humans
00:03:31.28 is called Homo erectus.
00:03:33.24 Homo erectus evolved around 2 million years ago,
00:03:39.02 and then after the origin of Homo erectus in Africa,
00:03:42.24 Homo erectus spread across Eurasia
00:03:47.17 and, indeed, shown here are some of the
00:03:49.21 oldest fossils of Homo erectus,
00:03:52.18 dated to as early as 1.9 million years ago (MYA) in Indonesia.
00:04:00.15 And this species was very successful,
00:04:03.14 lasting to as recently as 25,000 years ago
00:04:06.17 in Southeast Asia.
00:04:09.08 A very interesting recent finding was
00:04:11.20 a set of fossils identified on the island of Flores,
00:04:14.26 which is within Indonesia,
00:04:17.25 and these fossils actually show some characteristics
00:04:21.22 that look very similar to Homo erectus,
00:04:24.19 and for that reason it was proposed that
00:04:27.09 this species may have directly evolved
00:04:30.23 from a Homo erectus ancestor
00:04:33.20 that arrived on that island
00:04:36.07 about 1 million years ago
00:04:37.28 and then evolved in isolation.
00:04:39.25 And two of the very unique features of this species
00:04:42.17 is that they were very short, so again,
00:04:46.01 about the same size as Lucy, around 3 feet tall,
00:04:50.15 and secondly, that they had tiny brains.
00:04:53.14 And there's been a lot of debate about
00:04:55.01 whether this is an adaptation or in fact a pathology,
00:04:58.09 and there's still a lot of research being done,
00:05:01.03 but what was clear is that there were multiple species
00:05:04.01 outside of Africa
00:05:05.29 within the past 2 million years.
00:05:08.20 So now let's move on to the origins of
00:05:10.15 Homo neanderthalensis and Homo sapiens.
00:05:13.12 There's some question about the species preceding
00:05:16.28 Neanderthal and Homo sapiens.
00:05:19.17 Some say that it was heidelbergensis,
00:05:22.04 but there's debate about that.
00:05:24.15 However, what is clear is that the Neanderthals species
00:05:28.10 arose somewhere within the past 300,000-400,000 years,
00:05:32.15 and Homo sapiens arose within the past 200,000 years.
00:05:38.04 And this is a fossil from Neanderthals,
00:05:40.29 we can see a few features such as
00:05:44.02 the double arched and very wide brow ridges,
00:05:47.08 a broad nose,
00:05:48.28 a very large brain size,
00:05:50.27 and a retromolar space,
00:05:52.21 and in fact these species were very robust.
00:05:55.16 The males would have been over 6 feet tall,
00:05:57.15 they had very big bones,
00:05:59.19 and they had rather big brains.
00:06:02.20 In fact, here are some reconstructions of Neanderthal.
00:06:06.28 We have the old reconstruction
00:06:09.03 and then the more recent one as well.
00:06:12.11 So, anatomically modern humans, Homo sapiens sapiens,
00:06:16.06 arose approximately 200,000 years ago.
00:06:19.02 In fact, here these red dots
00:06:21.09 are representing locations where fossils have been found
00:06:24.11 of anatomically modern humans,
00:06:26.27 and the oldest fossil is
00:06:28.22 dated to around 150,000-195,000 years ago,
00:06:32.19 in Southern Ethiopia.
00:06:36.23 We also see evidence of early modern human behavior
00:06:40.10 dated to 70,000 years ago,
00:06:42.11 or even as old as 120,000 years ago,
00:06:45.16 in caves in south Africa
00:06:47.13 and also some from east Africa as well.
00:06:51.05 So after modern humans arose in Africa within the past 200,000 years,
00:06:55.08 one or a few small groups of individuals
00:06:57.25 migrated across the rest of the globe
00:07:00.11 within the past 50,000-100,000 years.
00:07:03.23 Indeed, we think that Europeans...
00:07:07.15 there were no people in Europe, actually,
00:07:09.06 until about 40,000 years ago,
00:07:11.13 and then modern humans crossed the Bering Straits
00:07:14.15 and went into the Americas
00:07:16.28 within the past 30,000 years.
00:07:19.05 The earliest migration event was actually into Australo-Melanesia,
00:07:23.11 dated to about 40,000-60,000 years ago.
00:07:26.14 And then we have much more recent migration events,
00:07:29.03 such as into the Pacific Islands,
00:07:31.12 within the past few thousand years.
00:07:34.11 Now, interestingly,
00:07:36.16 when modern humans migrated out of Africa
00:07:39.08 within the past 50,000-100,000 years,
00:07:42.05 they would have run into Neanderthals,
00:07:44.10 in fact they overlapped in their distribution.
00:07:47.08 So shown here is the distribution of Neanderthals,
00:07:50.22 and the modern humans who lived at that time
00:07:52.25 were referred to as Cro-Magnon,
00:07:55.17 and in fact we did not see anatomically modern humans
00:07:59.09 in this region, in Europe, until about 40,000 years ago.
00:08:03.03 They would have been in the Middle East a little bit earlier,
00:08:05.23 but it appears they overlapped
00:08:08.18 for about at least 10,000 years with Neanderthals.
00:08:12.13 And as we'll discuss later,
00:08:13.27 there is some evidence that there could have been actual admixture
00:08:17.05 between Neanderthal and anatomically modern humans
00:08:20.18 during that time.
00:08:22.26 So now I want to discuss the evolutionary forces
00:08:25.27 that influence the patterns of genetic variation
00:08:28.08 that we see today.
00:08:30.04 And these include mutation,
00:08:32.14 genetic drift,
00:08:33.29 migration,
00:08:35.09 and natural selection.
00:08:37.16 So let's first introduce some terminology.
00:08:40.05 The gene pool refers to the set of all genomes
00:08:42.25 in a specified population,
00:08:44.10 and here we have an example from a population of warthogs.
00:08:47.22 So where we have at a single genetic locus
00:08:51.03 two alleles, big B or little b,
00:08:54.17 and here's an example of an individual
00:08:56.11 who is homozygous for the big B allele,
00:08:59.07 and an individual homozygous for the little b allele,
00:09:02.12 and here's an individual who is heterozygous
00:09:05.08 for big B and little b.
00:09:07.12 And together, the set of alleles in that population
00:09:10.19 represents the gene pool.
00:09:13.28 So when we are doing population genetics analyses,
00:09:16.25 we can't actually go out and look at every genotype
00:09:21.00 for every individual in the population,
00:09:23.14 that would be unfeasible.
00:09:25.13 So what we typically do is to
00:09:26.23 infer frequencies by estimating them
00:09:30.10 from a random sample.
00:09:32.25 So in population genetics
00:09:35.01 generation, each new individual
00:09:37.16 is viewed as drawing from a set of gametes
00:09:39.20 with alternative alleles,
00:09:41.08 so let's use an example here
00:09:43.01 in which we have a set of marbles in a bowl.
00:09:46.05 And initially, we have a distribution of
00:09:51.26 60 of the white marbles
00:09:54.13 relative to 40 of the green marbles,
00:09:56.27 and these, the white and the green,
00:09:58.08 are representing different alleles.
00:10:00.14 So let's say that we're gonna pick...
00:10:02.04 we're gonna reach into this bag
00:10:04.04 and we're gonna randomly draw out
00:10:06.09 another hundred of these marbles.
00:10:09.01 And now in the next generation
00:10:10.26 we have 80 of the white and we have 20 of the green.
00:10:15.02 We're gonna reach back in,
00:10:16.01 we're gonna grab another set of a hundred,
00:10:18.09 and now in the next generation
00:10:20.15 we have 100 of the white alleles and 0 of the green.
00:10:26.08 And this is a demonstration of
00:10:27.15 how we get changes in allele frequency over time.
00:10:31.25 Allele frequencies will also change over time
00:10:34.23 due to genetic drift,
00:10:36.21 which is defined as random fluctuations
00:10:39.01 of allele frequencies from generation to generation,
00:10:42.03 simply due to chance.
00:10:44.19 So as we see, sometimes things could happen,
00:10:47.16 like these bugs are getting squashed,
00:10:50.00 and that's gonna change, perhaps,
00:10:52.07 the allele frequency in the next generation.
00:10:55.19 Here's another example from some lady bugs,
00:10:58.23 and we can see that, perhaps,
00:11:01.03 in the next generation, just by chance,
00:11:03.10 we're gonna see more of these ladybugs
00:11:04.29 with the dark colors,
00:11:06.12 or we might see more that are with the medium colors and dots.
00:11:10.16 And the fact is that drift is just an inevitable fact of life.
00:11:16.15 I also want to define what we mean by neutral evolution.
00:11:20.08 So we define a selectively neutral allele
00:11:22.10 as one that does not affect reproductive fitness of individuals
00:11:25.20 who carry that allele,
00:11:27.20 so it's frequency in the population
00:11:29.25 changes by chance or genetic drift alone.
00:11:32.18 And here we have an example:
00:11:35.04 this is just a substitution
00:11:37.22 in the third position of the codon,
00:11:41.02 and when we have substitutions
00:11:44.09 of nucleotides in the third position,
00:11:46.20 very typically they result in a silent or synonymous change.
00:11:51.05 So here there's been a substitution,
00:11:53.00 but there's no change in the amino acid;
00:11:55.02 it remains as valine.
00:11:57.26 So the rate at which genetic drift occurs
00:12:00.01 is going to inversely proportional to the population size, N,
00:12:03.23 and it's going to be very fast in small populations.
00:12:06.27 And here's an example that we can look at
00:12:08.23 based on computer simulation.
00:12:11.20 So let's assume here that we're looking at a single locus
00:12:15.15 and it has two alleles
00:12:18.06 that are at 50% frequency each,
00:12:21.25 as we can see here.
00:12:23.22 We have a sample size of 25,
00:12:27.06 and we're going to do the simulation
00:12:29.03 over 80 generations.
00:12:31.14 Now, each of these lines here
00:12:34.03 represents a different simulation,
00:12:36.27 and what we can see is that
00:12:38.23 over time alleles are either going to
00:12:44.02 be lost from the population
00:12:46.08 or they're going to reach fixation,
00:12:48.17 which means that they go to 100% frequency.
00:12:52.10 And the rate at which this occurs
00:12:54.00 is going to depend on the sample size.
00:12:56.09 So in a small sample it's gonna be very rapid,
00:12:59.19 but in this example where we have a larger sample, now N=300,
00:13:03.26 you can see that it just takes more time.
00:13:05.23 There's not as much genetic drift occurring.
00:13:08.19 Now, the end result is gonna be the same,
00:13:10.15 it just takes more time.
00:13:14.09 The change in allele frequency also is going to depend
00:13:17.27 on the initial allele frequencies.
00:13:19.20 So in this particular case,
00:13:21.05 we've now changed the starting frequency:
00:13:23.20 it's not 50%, it's now 10%.
00:13:27.06 And you can see that there's much more
00:13:29.28 probability of loss of the allele in this case,
00:13:34.11 and here we have just one of the alleles reaching fixation.
00:13:42.08 So again, in this particular case,
00:13:44.05 about 1 out of 10 will eventually become fixed,
00:13:47.14 or reach 100% frequency.
00:13:51.09 Now here's an example from a large population.
00:13:54.01 It'll take longer for this to occur,
00:13:56.02 but the proportion of alleles are gonna be
00:13:58.12 roughly the same,
00:13:59.29 so again roughly 1 out of 10 will go to fixation,
00:14:03.06 it's just gonna take longer.
00:14:05.16 Other important terms in population genetics
00:14:07.26 are bottleneck and founder effects,
00:14:10.08 and this is because genetic drift
00:14:11.23 has a large effect on allele frequencies
00:14:14.10 when a population originates
00:14:16.05 via a small number of people from a larger population.
00:14:19.16 So here we have an example of a bottleneck,
00:14:22.10 and what a bottleneck means is that
00:14:24.01 there's been a decrease in population size
00:14:26.21 at some time in the past.
00:14:28.14 So you can think of it as a population crash.
00:14:31.10 And what happens when the population is very small,
00:14:34.28 you're going to have a higher rate of genetic drift,
00:14:37.12 and we can see here that these alleles,
00:14:39.20 which are represented by the different colors,
00:14:42.00 have shifted from what we're seeing
00:14:44.18 back at this earlier time.
00:14:46.25 Now we go through the bottleneck,
00:14:48.19 and now we're seeing predominantly
00:14:50.07 these white and black alleles.
00:14:53.09 Another example we can look at is a founder event,
00:14:57.20 which is sort of a special case of a bottleneck event.
00:15:00.11 And in this case it's where a population, a small population,
00:15:05.03 breaks off from the larger population,
00:15:07.25 and again there's going to be increased genetic drift
00:15:10.26 in this initially small population
00:15:13.12 and here, by chance,
00:15:15.05 we just happened to see more of these dark blue
00:15:18.12 and light blue alleles.
00:15:21.09 The pattern of variation that we see
00:15:22.23 in the human genome
00:15:24.09 is also dependent on the effective population size,
00:15:27.17 which we distinguish as capital N sub e.
00:15:32.10 And the definition of the effective population size
00:15:35.10 is the number of breeding individuals in a population.
00:15:38.19 So estimates of Ne
00:15:40.17 are most strongly influenced by population sizes
00:15:43.07 when they're at their smallest,
00:15:45.10 and it could take many generations
00:15:47.02 to recover from a bottleneck event.
00:15:49.11 So estimates of Ne in modern populations
00:15:51.21 reflect the size of the population
00:15:53.20 prior to population expansion.
00:15:56.22 Pretty consistently, studies of nuclear sequence diversity in humans
00:16:00.24 have estimated an effective population size
00:16:03.15 of about 10,000.
00:16:05.19 Now, by contrast, if we look at Chimpanzees,
00:16:08.29 the estimate is closer to 35,000.
00:16:12.14 And so what that means is that
00:16:14.01 humans have undergone a bottleneck
00:16:16.18 sometime during their evolutionary history.
00:16:19.22 So the pattern of genomic variation
00:16:21.25 that we see in modern populations today
00:16:24.00 is a reflection of our evolutionary and demographic history.
00:16:27.14 So how much do we differ?
00:16:29.17 Well, identical twins
00:16:31.27 have no differences at the nucleotide level.
00:16:35.06 If we compare unrelated humans,
00:16:36.29 we differ at about 1 out of 1,000 nucleotide sites.
00:16:41.12 And if we compare humans to our closest genetic relative, the Chimpanzee,
00:16:45.02 we differ at about 1 out of 100 sites.
00:16:47.29 So, as a whole, our species is very similar,
00:16:50.27 and that simply reflects our recent common ancestry
00:16:54.05 from Africa within the past 100,000 years.
00:16:57.06 But when you consider that there are
00:16:58.27 over 3 billion DNA bases in the genome,
00:17:02.02 that results in 3 million differences
00:17:04.16 between each pair of genomes,
00:17:06.05 more than enough to generate the diversity
00:17:08.29 that will make each of us unique.
00:17:12.02 Now I want to introduce a statistic
00:17:14.13 that we typically use to look at how much variation
00:17:17.06 there is among populations,
00:17:20.01 and this is referred to as an Fst statistic.
00:17:24.00 And it's simply looking at the proportion of genetic variation
00:17:27.03 that is within populations,
00:17:29.06 relative to that which is between populations.
00:17:32.18 Fst can be measured based upon heterozygosity,
00:17:37.20 and heterozygosity is simply a measure of genetic variation,
00:17:41.26 which is very simply calculated as
00:17:44.15 1 minus the sum of the allele frequencies squared.
00:17:49.09 And so once we calculate
00:17:51.26 the heterozygosity for each locus,
00:17:53.29 we can look at the average,
00:17:55.23 and we can look at the average within a subpopulation,
00:17:58.03 or in the total combined population.
00:18:00.29 Now, just as an example,
00:18:03.15 if we were to see here that
00:18:06.22 in the case of Fst = 1,
00:18:09.12 it means that there is no overlap at all in the allele frequencies.
00:18:13.15 So we can see that in population 1 they have all A's,
00:18:16.13 and in population 2 they have all B's.
00:18:19.15 And in the case of Fst = 0,
00:18:22.18 there is complete similarity,
00:18:26.08 so here we see exactly the same number
00:18:28.13 of A alleles and exactly the same number of B alleles.
00:18:32.01 And then here's an intermediate case
00:18:33.29 where we have about 0.11, 11%,
00:18:39.07 showing that there's just a small amount of differentiation
00:18:43.04 between these two populations.
00:18:46.09 So what do we see in humans?
00:18:47.29 Well, the average Fst between human populations
00:18:51.04 is about 15%,
00:18:53.15 and what that means is that the majority of genetic variation
00:18:56.04 is found within a population,
00:18:59.07 and only about 15% of the genetic diversity
00:19:02.08 differs between populations.
00:19:04.23 Again, this is reflecting our recent common ancestry in Africa,
00:19:09.00 within the past 50,000-100,000 years.
00:19:14.13 Now, interestingly,
00:19:16.09 if we were to do this calculation from Chimpanzee populations,
00:19:19.08 we see that the value is around 32%,
00:19:22.15 so there's actually a lot more differentiation
00:19:25.04 among Chimpanzee populations
00:19:27.07 than among human populations,
00:19:29.18 again reflecting our overall close genetic similarity to each other.
00:19:36.19 So I now want to talk about the
00:19:38.04 different sources of DNA that we use
00:19:40.04 to reconstruct human evolutionary history.
00:19:43.01 One source of DNA is
00:19:45.29 that which is present in the nuclear genome
00:19:48.06 that's located in the nucleus of the cell.
00:19:51.03 And there's another type of genome
00:19:53.20 which is present in the mitochondria of the cell,
00:19:56.15 and the mitochondria is the energy-producing organelle.
00:20:02.13 So what is the difference between these different genomes?
00:20:06.03 Well, the nuclear genome
00:20:08.09 consists of 22 autosomal pairs of chromosomes
00:20:12.26 and then the sex chromosomes,
00:20:14.15 XX for females and XY for males.
00:20:17.27 The nuclear genome is about 3.4 billion bases in size,
00:20:22.02 and it consists of about 20,000 coding genes.
00:20:25.10 It's inherited from both parents,
00:20:27.21 but it also undergoes extensive recombination each generation.
00:20:32.07 But, one of the reasons it's useful is that there's
00:20:34.18 so many different locations where we can study variation,
00:20:38.08 given that there are 3 billion nucleotides,
00:20:41.02 it's just a little bit more difficult to trace them back
00:20:43.29 to a single common ancestor.
00:20:46.20 By contrast, the mitochondria DNA genome
00:20:50.21 is very small, it's only about 16,000 nucleotides in size,
00:20:55.14 and it's circular,
00:20:57.17 and it's passed on only through the maternal lineage.
00:21:00.19 There's also no recombination
00:21:02.17 and it has a very high mutation rate.
00:21:05.00 All of these features make it very useful
00:21:07.01 for tracing evolutionary history.
00:21:09.27 So let me give you another example of what I'm referring to.
00:21:13.12 The mitochondrial DNA is inherited through the maternal lineage,
00:21:17.05 whereas the nuclear DNA is inherited from both parents.
00:21:22.08 So if we were to trace back from a present day individual,
00:21:25.26 they will have inherited their nuclear genome
00:21:28.20 from their parents,
00:21:30.17 their parents would have inherited from their set of parents,
00:21:33.28 and then their set of parents, and so on.
00:21:36.15 So we can trace it back to a large number of ancestors.
00:21:39.16 But by contrast, if we're tracing back mitochondrial DNA lineages,
00:21:44.00 we can see that they're only passed on
00:21:46.25 through the maternal lineage,
00:21:49.10 so they're essentially inherited from a single lineage.
00:21:52.03 We can trace them back to a single common female ancestor,
00:21:56.01 and that's why they're been very useful
00:21:57.29 for human evolutionary genetics studies.
00:22:00.21 So for example, if we were to consider
00:22:02.26 these dots to be mitochondrial DNA lineages,
00:22:06.20 and let's start at generation 11 at the bottom,
00:22:10.12 shown by the red dots,
00:22:12.06 and imagine those are different mitochondrial DNA sequences
00:22:15.00 from different individuals.
00:22:17.10 At some time in the past, these two individuals, for example,
00:22:22.06 coalesced back to a common ancestor,
00:22:24.26 and then this group coalesces back to a common ancestor here,
00:22:29.29 and ultimately they all coalesce back
00:22:32.20 to a single common ancestor.
00:22:35.03 Now, in the popular literature,
00:22:36.22 the single common ancestor for mitochondrial DNA
00:22:39.04 is often referred to as "mitochondrial Eve",
00:22:42.21 but one thing to remember is that
00:22:45.17 Eve was not alone, she lived within a population,
00:22:49.06 as we can see here by the other colors.
00:22:51.22 But those lineages just never made it
00:22:54.22 down to the present day.
00:22:57.25 So this is a phylogenetic tree
00:23:00.11 constructed by sequencing mitochondrial DNA
00:23:03.10 whole genome lineages
00:23:05.02 from ethnically diverse individuals.
00:23:07.19 So each individual actually represents
00:23:10.29 a branch on this tree,
00:23:13.02 and if two individuals are very closely related to each other,
00:23:16.05 they'll be very close to each other
00:23:19.01 in the tree.
00:23:21.03 So one of the first things you can see
00:23:22.19 using Chimpanzee as an outgroup
00:23:25.01 is that all modern human lineages
00:23:27.25 coalesce at about 170,000 years ago,
00:23:31.12 and so that corresponds very well with the
00:23:33.05 time of origin of anatomically modern humans.
00:23:36.23 So another thing that we can see is that
00:23:39.25 all of the oldest genetic lineages
00:23:42.26 are from African individuals.
00:23:45.22 We can also see that
00:23:48.12 the very oldest lineages
00:23:50.15 are from the San and the Mbuti pygmy hunter-gatherers,
00:23:54.28 and then the more recent lineages
00:23:57.13 are from non-African populations.
00:24:00.01 And that is a pattern that's very consistent
00:24:02.17 with the model of a recent African origin
00:24:05.12 of modern humans.
00:24:07.23 Now, another way that we can compare mitochondrial DNA sequences
00:24:11.21 is to simply count up the number of sites
00:24:14.04 at which they differ when we compare any pair of sequences.
00:24:17.23 And when we do this,
00:24:19.09 we observe that
00:24:22.11 any two African lineages will differ from each other
00:24:25.03 at many more sites than any two non-African lineages.
00:24:29.06 And again, that means that there has been more time
00:24:32.02 for variation to accumulate in Africa,
00:24:34.16 and is consistent with an African origin
00:24:37.08 of modern humans.
00:24:39.20 When we sequence the mitochondrial DNA lineages,
00:24:42.21 we can classify them as haplotypes,
00:24:45.10 and those haplotypes belong to
00:24:47.16 larger subsets of haplogroups.
00:24:50.01 You can think of a haplotype as simply
00:24:52.14 the arrangement of genetic variants along a chromosome,
00:24:55.19 or in the case of the mitochondrial DNA
00:24:57.22 there's just a single genome,
00:24:59.14 so it's really just the different nucleotide differences
00:25:02.27 amongst different mitochondrial DNA lineages.
00:25:06.24 And one of the first things that you can note is that
00:25:09.26 there are different haplogroups
00:25:11.29 in different regions of the world.
00:25:13.19 So here are some that seem to be pretty specific to Africa,
00:25:16.20 but are also present in some regions
00:25:18.20 where there may have been some gene flow
00:25:20.20 from Africa.
00:25:22.21 Then we have others that may be more common in Europe,
00:25:25.12 or in east Asia,
00:25:28.18 or in the Americas.
00:25:30.19 And for that reason,
00:25:32.11 mitochondrial DNA can be very useful for
00:25:34.11 tracing recent human migration events.
00:25:38.13 Now, by contrast,
00:25:40.02 the Y chromosome is also inherited with no recombination,
00:25:45.14 and so it can also be very useful for tracing back
00:25:48.01 through the male lineages.
00:25:50.16 And here is a phylogeny constructed from Y chromosome variation,
00:25:55.07 and as with the mitochondrial DNA,
00:25:58.08 what we see is that the oldest lineages
00:26:01.19 are specific to Africans,
00:26:04.02 and the more recent lineages
00:26:06.05 are found predominantly in Non-Africans,
00:26:08.13 although we do see some in Africans as well.
00:26:11.25 Again, this is consistent with the recent African origin of modern humans.
00:26:18.14 We can also look at Y chromosome haplogroups,
00:26:22.09 and one of the things that's a little bit different
00:26:24.04 is you can see that they're a bit more differentiated
00:26:26.16 between geographic regions.
00:26:29.03 So for example,
00:26:30.24 here we just see haplogroups that are in blue,
00:26:34.04 and we see very distinct haplogroups
00:26:36.20 in the Americas, shown in purple.
00:26:39.26 And one of the reasons for that may have to do with
00:26:43.08 sex-biased migration,
00:26:46.01 that you may have, for example,
00:26:47.16 one male traveling long distances.
00:26:50.06 And it may also have to do with patterns of mating structure.
00:26:54.20 So for example, in some populations or ethnic groups,
00:26:57.23 you may have one male who has many different wives,
00:27:01.05 and because of that the effective population size of the Y chromosome
00:27:07.01 is actually smaller than the mitochondrial DNA,
00:27:09.28 and we tend to get more genetic differentiation
00:27:12.27 around the world.
00:27:15.07 So now I want to talk about analyses of ancient DNA,
00:27:18.27 for example, in this case from Neanderthal,
00:27:22.12 and these are some images of scientists
00:27:25.20 working on a Neanderthal fossil.
00:27:29.10 And this type of analysis is very challenging
00:27:32.01 for a number of reasons.
00:27:33.25 One is that DNA which is that old,
00:27:38.04 on the order of say 30,000 years old
00:27:40.10 to even 100,000 years old,
00:27:42.06 is going to be highly degraded,
00:27:44.24 and if there's any contamination
00:27:46.25 with modern human DNA,
00:27:49.02 that is much more likely to amplify
00:27:51.19 than the old degraded DNA
00:27:54.01 from the archaic species,
00:27:56.21 so one has to be extremely careful when analyzing this DNA.
00:28:01.03 Now, more recently,
00:28:02.24 there was a pinky finger bone
00:28:05.07 identified in a cave in Siberia
00:28:07.22 from a region called Denisova,
00:28:10.11 so it's referred to as the Denisova
00:28:13.21 or Denisovan genome.
00:28:16.11 Here I'm presenting a phylogenetic tree
00:28:18.29 based on mitochondrial DNA variation
00:28:21.24 comparing modern humans, shown in blue here,
00:28:26.09 to Neanderthals shown in red,
00:28:29.01 and the Denisova individual shown in yellow.
00:28:32.23 And what we can see is that the
00:28:34.17 time to most recent common ancestry in humans,
00:28:37.08 as we've already discussed,
00:28:39.00 is about 200,000 years ago.
00:28:41.13 The time to most recent common ancestry
00:28:43.14 between humans and Neanderthals
00:28:46.01 is about 500,000 years ago,
00:28:48.13 for the mitochondrial DNA lineages.
00:28:51.03 And the time to most recent common ancestry
00:28:53.20 with the Denisova mitochondrial lineages
00:28:57.08 is about 1 million years ago.
00:29:00.05 So this is demonstrating a couple of things.
00:29:02.20 From the mitochondrial DNA perspective,
00:29:05.07 there's no evidence of any admixture
00:29:07.13 with anatomically modern humans.
00:29:10.02 The Neanderthal sequences are clearly
00:29:12.18 very distinct from modern humans.
00:29:14.28 It's also showing you that there was another species, Denisova,
00:29:18.15 that appears to be distinct from the Neanderthals,
00:29:21.07 and they diverge even earlier than Neanderthals
00:29:24.09 from modern humans.
00:29:26.21 So if we were to compare pairwise nucleotide diversity,
00:29:31.01 for example,
00:29:33.02 among anatomically modern humans shown in blue,
00:29:35.24 you can see that there's not a lot of diversity,
00:29:38.15 as expected considering that
00:29:40.13 we all have a very recent common ancestry.
00:29:43.04 If you compare the modern human mitochondrial genomes to Neanderthal,
00:29:48.03 you can see that they're more divergent,
00:29:50.07 as expected, given that the mitochondrial DNA lineage
00:29:54.04 diverged about 500,000 years ago.
00:29:57.02 If we compare to the
00:29:59.03 Denisovan mitochondrial DNA lineage,
00:30:01.10 they're even more divergent.
00:30:04.04 And then if we compare to Chimpanzee,
00:30:06.14 of course as expected,
00:30:08.11 given that they diverged at least 5 million years ago,
00:30:11.14 they are the most different in terms of sequence variation.
00:30:15.13 Now, several years ago
00:30:18.13 there was a draft sequence produced of
00:30:21.20 the Neanderthal genome using next-generation sequencing technology.
00:30:25.25 And this was an absolutely amazing feat,
00:30:28.17 but at the time they had very low coverage,
00:30:31.07 meaning that any particular region of the genome
00:30:33.19 was sequenced only about once or twice.
00:30:36.20 Now, more recently,
00:30:38.07 as the technology has improved,
00:30:40.05 they've gotten much better coverage of the Neanderthal sequence,
00:30:43.04 and quite recently they now have a 30-fold coverage,
00:30:46.22 meaning that on average most sites
00:30:49.03 will have sequenced 30 times.
00:30:51.22 And so you'll have a much better accuracy
00:30:54.23 when determining nucleotide variation.
00:31:01.07 So, when the Neanderthal genome
00:31:03.25 was compared to the human genome,
00:31:06.11 what you can do is first
00:31:08.10 look at how much divergence has occurred
00:31:11.02 since modern humans differentiated from Chimpanzees
00:31:15.10 within the past 6.5 million years.
00:31:18.12 And you can look at the divergence
00:31:20.24 that has occurred specifically in the human lineage
00:31:24.06 since they diverged from Neanderthal,
00:31:26.21 and they've only accumulated
00:31:29.07 about 8% of this total divergence.
00:31:34.08 And so the estimate of the time of population divergence
00:31:38.06 between humans and Neanderthals
00:31:40.15 is about 400,000 years ago.
00:31:43.09 Furthermore, it has been estimated that
00:31:45.24 there may have been a small amount of admixture
00:31:48.16 between Neanderthals and anatomically modern humans,
00:31:52.01 as shown by this red arrow here.
00:31:54.18 So the estimated amount of admixture is about 1-2%,
00:32:00.15 of the modern human genome,
00:32:02.17 may be of Neanderthal ancestry.
00:32:05.03 But what is of interest is to note that
00:32:07.24 this is only present in Non-Africans.
00:32:10.13 It is not present in African genomes.
00:32:13.05 And so what we can infer from that is
00:32:15.16 that this admixture event probably occurred
00:32:18.25 before modern humans spread across the globe.
00:32:22.01 It may have occurred, for example, in the Middle East,
00:32:24.28 and that's why we're seeing it present in all Non-Africans,
00:32:29.18 and we don't see it at all in Africans.
00:32:32.15 Now, more recently, there has been
00:32:34.22 whole genome sequencing of the Denisovan individual,
00:32:39.20 and what that has shown is that
00:32:42.09 the Denisovan species, or this individual,
00:32:45.15 appears to have diverged from modern day humans
00:32:48.13 around 800,000 years ago,
00:32:51.09 consistent with what we saw from the mitochondrial DNA.
00:32:55.21 They also observed low levels of heterozygosity in Denisova,
00:32:59.21 suggesting that they may have had
00:33:01.19 a small population size.
00:33:04.06 Additionally, when a phylogenetic tree
00:33:07.24 was constructed from the nuclear DNA variation,
00:33:11.13 they could see that the modern humans
00:33:15.11 tend to cluster together,
00:33:17.09 and as we expect they're divergent
00:33:19.01 from the Denisova and the Neanderthals.
00:33:21.29 The Neanderthals tend to cluster together,
00:33:24.06 so they're clearly divergent from Denisova.
00:33:27.03 But what's interesting is if you look at how much
00:33:31.01 variation there is amongst the modern humans,
00:33:34.11 as indicated by the length of these lineages,
00:33:38.06 and then you compare that to Neanderthals,
00:33:40.14 which have very short branches.
00:33:43.06 What that suggests is
00:33:44.28 that there was not a lot of genetic variation
00:33:47.09 amongst the Neanderthals,
00:33:49.23 and therefore they may have undergone a bottleneck,
00:33:52.11 so they might have undergone a population crash
00:33:54.20 at some point in the past.
00:33:57.07 So in summary,
00:33:59.04 what we can see is that
00:34:01.23 Homo erectus left Africa
00:34:04.05 within the past 2 million years,
00:34:06.28 and spread throughout Eurasia,
00:34:09.09 giving rise, possibly,
00:34:11.09 to species like Homo floresiensis,
00:34:14.17 and surviving until quite recently,
00:34:17.12 as recently as around 25,000 years ago.
00:34:20.28 Then we have other species like Neanderthal and Denisovans,
00:34:27.02 who may have originated from a different species,
00:34:30.07 such as heidelbergensis,
00:34:33.10 and they differentiated sometime
00:34:36.12 around 600,000 or 700,000 years ago in the case of Denisova,
00:34:39.29 or in Neanderthals around 400,000 years ago.
00:34:43.05 And then we have the modern human lineage,
00:34:46.11 Homo sapiens,
00:34:49.00 which arose around 200,000 years ago
00:34:51.07 and spread out of Africa.
00:34:53.21 And when they did so,
00:34:55.02 they would have encountered these other species,
00:34:57.09 and there may have then been low levels of gene flow.
00:35:01.20 And in fact for the case of the Denisovan genome,
00:35:03.23 it appears that the gene flow
00:35:05.26 was predominantly with populations from Oceania,
00:35:10.01 implying that this admixture
00:35:12.17 may have occurred in a different location and a different time.
00:35:16.00 Now, we still don't know exactly
00:35:18.05 how much admixture there may have been
00:35:20.12 between archaic species
00:35:22.23 and modern humans in Africa,
00:35:25.01 but there's some preliminary data suggesting that
00:35:27.10 this has occurred there as well.
00:35:29.14 The problem is that the fossils don't preserve as well in Africa,
00:35:32.19 so we don't have any DNA sequences
00:35:34.26 from archaic lineages in Africa at this point.
00:35:40.01 So in conclusion,
00:35:41.18 Africa has the most genetic diversity in the world.
00:35:44.15 Human dispersions out of Africa
00:35:46.11 populated the entire world,
00:35:48.15 and we are the last of a series of hominin dispersal events
00:35:51.14 out of Africa.
Genetics of Morphology
Concepts: Natural selection, fitness, genotype determines phenotype, convergent evolution of color pattern in mice
- Duration: 36:12
- Downloads
- Subtitles
- Transcript
00:00:00.04 Hi, my name is Hopi Hoekstra and I'm a professor at Harvard
00:00:03.10 University. And in this second segment, what I would like to do is
00:00:06.15 tell you a story about the genetic basis of evolutionary
00:00:09.22 change. And in particular, we're going to focus on the story
00:00:12.06 of a morphological trait. I'm going to tell you this story in the context
00:00:17.15 of making links between environment, phenotype, and
00:00:20.08 genotype. In particular, I'm going to tell you today a three-part story. The first
00:00:24.18 part of the story, I'm going to tell you about a role for natural
00:00:28.09 selection in driving differences and traits in a natural population.
00:00:31.14 And then I'm going to take you from the field into the lab,
00:00:34.11 where we've been doing work to understand the genetic basis
00:00:37.06 or the genes that control this phenotypic difference. And it's
00:00:40.12 not just that we want to know the genes, but I'll tell you
00:00:42.07 about how changes in these genes through development
00:00:44.25 actually produce variations in the phenotype. And then once we
00:00:48.02 made all the links, then we'll have a more complete understanding
00:00:50.14 of the process of adaptation. And here's where I think things can get
00:00:53.24 really fun. Because we can go back out into the wild
00:00:56.22 and ask how these traits evolved in natural populations. So let's
00:01:00.10 start today at the level of phenotype. So the phenotype I'm going to
00:01:03.23 tell you about is one that we've been studying in my group for almost
00:01:06.03 a decade. And that is color variation. So why do we study color?
00:01:10.25 Well, color is one of the primary ways in which organisms
00:01:14.11 interact with their environment. And it varies tremendously
00:01:18.04 between species, and it can also vary between species. And in
00:01:22.04 particular, we know at least in some cases, even small changes
00:01:25.03 in color difference can have a huge impact on fitness.
00:01:27.22 The ability of organisms to reproduce and survive in the wild.
00:01:31.03 So here I've just given you a number of examples of how
00:01:34.21 color is used. It can be used, for example, in terms of reproduction
00:01:38.21 in that it can be used for mate choice. So we have the canonical example of
00:01:41.29 the peacock's tail. But flowers also use color to attract pollinators
00:01:46.26 which is the way that they reproduce. Color can also be
00:01:49.20 used not to attract other species or other individuals,
00:01:52.18 but it can be a way to warn them of let's say a distasteful
00:01:56.29 poison. As is the case in these poison frogs.
00:02:00.29 Now, no good biological story comes without having
00:02:04.16 cheaters. So we also have mimics, those species that
00:02:08.14 are themselves not toxic, but mimic those that are toxic.
00:02:13.09 And thereby are also avoided by predators. But by
00:02:17.00 far, color is used most commonly in terms of camouflage or
00:02:21.23 in terms of crypsis. So that's what I'm going to focus on today.
00:02:25.00 In addition to being ecologically relevant, the other reason we
00:02:29.23 study color is because we can rely on nearly a decade's worth of work
00:02:34.22 by geneticists who have been tracking down genes involved
00:02:37.16 in spontaneous mutations that occur in laboratory populations
00:02:40.28 of mice. So here what I've done is I've made a list, which again
00:02:44.04 I don't expect you to read, but just to appreciate that we know a lot
00:02:47.25 of genes. And if you mutate that gene, it's going to have an effect on
00:02:51.08 color. And here are some of the mutant color differences
00:02:55.00 that have spontaneously arose in laboratory colonies of
00:02:57.26 mice. So in some sense, we have a little bit of a headstart because
00:03:00.26 we know a number of what I'll refer to as candidate genes,
00:03:03.29 genes that contribute to color differences in mammals, but may
00:03:08.05 also contribute to natural variation in color. Now unfortunately,
00:03:12.06 laboratory mice or their wild equivalents, while very common
00:03:15.14 in nature, for example, in our houses, vary a lot in laboratory
00:03:19.23 populations. They don't actually vary that much in the wild.
00:03:22.15 So, instead of studying these mice, we've decided to do
00:03:25.17 a study on a closely related group of mice, mice in the genus
00:03:29.20 peromyscus called deer mice, shown here in their slightly
00:03:32.21 flattened form. So this is a common way that mice are kept
00:03:36.25 in museum collections. And it nicely illustrates the variation in
00:03:40.24 color that I'm going to talk about today. So in this first image,
00:03:43.28 you can see that these deer mice vary tremendously in their
00:03:47.00 dorsal coat. They vary from almost completely white to
00:03:50.23 almost completely black. But they also vary not just in the
00:03:54.28 pigments and individual hairs on their dorsal coat, but they
00:03:57.19 also differ in the distribution of pigments across the body.
00:04:00.10 Or variation in color pattern, as shown here. So these candidate
00:04:05.04 genes that I showed you in the previous slide, many of those
00:04:07.23 are implicated in variation in dorsal color. But in fact,
00:04:11.04 we know very little about how patterns are made. So by studying
00:04:14.10 these mice, not only can we take advantage of this vast
00:04:17.00 range of variation in the color, but we also may learn something
00:04:20.02 new about color patterning. So you may have already noticed
00:04:24.09 that these slides were both taken by someone called Sumner back
00:04:28.07 in the 1920's. Well, this is Francis Sumner, shown here.
00:04:32.09 In his field regalia. This is actually the outfit that he would wear
00:04:36.16 when he would go out into the wild and trap mice. He's a
00:04:39.10 classic natural historian, he was associated with initially the
00:04:42.13 University of Michigan natural history museum. And he spent
00:04:46.29 a bulk of his career trying to answer the question of why
00:04:50.10 populations vary so much in nature. And to do this,
00:04:53.25 he would drive around the U.S. and catch mice, and document
00:04:57.20 variation in a number of traits, including color variation.
00:05:02.17 So Francis Sumner's favorite species of deer mouse, and
00:05:05.28 mine as well, is a particular species call peromyscus polionotus.
00:05:09.17 Peromyscus polionotus is often referred to as the old field
00:05:13.19 mouse. And that's because much of its range in the southeastern
00:05:16.27 U.S., as seen here, so Alabama, Georgia, South Carolina,
00:05:20.08 and Northern Florida. It occurs in these old fields, which are
00:05:24.13 really overgrown agricultural fields. Now throughout
00:05:28.13 most of its range, it may look to you like a typical mouse.
00:05:31.13 It's got a dark brown coat, a gray scruffy belly, and a striped
00:05:35.01 tail. But what's particularly interesting to us about these mice
00:05:38.19 is that they've recently invaded these sandbar islands and
00:05:44.15 sandy dune habitats on the Gulf coast of Florida, as well as
00:05:48.03 the Atlantic coast of Florida. So each one of these
00:05:51.16 numbers on this map refers to a different subspecies
00:05:54.13 of what I'll refer to as "beach mice," because the mice actually live
00:05:57.20 on the beach. Now, the first part of the story, I'm going to
00:06:00.29 focus on one of these subspecies. Here number 3.
00:06:04.04 And that is the Santa Rosa Island beach mouse. So let me show you
00:06:07.06 a picture of their habitat. So unlike their mainland counterparts
00:06:12.02 that live in dark loamy soils, these mice live on these beautiful
00:06:15.14 sandy islands off the Gulf coast of Florida. And here's a picture
00:06:20.03 of one of our field sites. So you'll notice that there are two
00:06:24.05 dramatic differences in habitat that these mice occupy.
00:06:26.25 So first, you can tell that the soil, in this case, this granulated
00:06:33.02 sand that's almost like walking on hills of granulated sugar.
00:06:36.07 It's much lighter in color than the dark loamy soils of the mainland
00:06:40.00 subspecies. But in addition, these beach habitats also have
00:06:45.17 much less vegetative cover, so these mice are exposed to
00:06:48.11 really high levels of predation. And I'll tell you more about
00:06:51.05 their predators in a minute. So it may not be surprising then,
00:06:55.01 when we go out and catch mice in these beautiful beaches,
00:06:58.08 the mice look different. So here's a picture of one of those beach
00:07:01.29 mice. And I should mention that this is not to scale.
00:07:04.02 So both of these mice are about the same size and they're about
00:07:06.23 the size of a ping pong ball. But what this slide does serve to illustrate
00:07:10.17 is the dramatic differences in pigmentation. So this particular
00:07:13.25 mouse, you can see is lacking pigment on its nose,on its sides, and if you could see the tail, it's also missing that
00:07:19.17 strong tail stripe. The other thing I want to mention to you
00:07:22.23 about this system is that we know something about the geological
00:07:25.14 age of these islands. They're about 6000 years old, which suggests
00:07:30.12 that the difference in coat color that you see here may have
00:07:33.17 evolved in just a few thousand years. So you may all be
00:07:37.24 thinking that this makes perfect sense, right? That these mice are
00:07:41.00 running around in these beautiful white sand beaches and having
00:07:43.04 a light color coat would make them more camouflaged.
00:07:46.15 And that's a great idea, but we wanted to actually prove that.
00:07:49.23 So as scientists, what we wanted to do is empirically
00:07:53.00 demonstrate that color matters for survival. So actually
00:07:56.07 do an experiment. We wanted to know how much it matters,
00:07:59.03 in other words, we want to estimate the strength of selection.
00:08:01.27 How favorable is it to actually match the background?
00:08:05.17 And then finally, we wanted to know the agent of selection.
00:08:07.25 Who's doing the selection? And in this case, who are the
00:08:10.17 predators? So first I want to tell you about the experiments we did
00:08:15.10 to try to make this link between color variation and the differences
00:08:18.25 in environment that these mice live in. And in particular, implicate a role
00:08:22.09 for natural selection. Now if I could do any experiment
00:08:25.13 in the world, here's what I'd love to do. I'd love to catch
00:08:28.25 let's say 100 light mice and 100 dark mice, maybe give them all
00:08:32.16 a tag. And let's say release half of them, equal numbers of
00:08:36.11 light and dark, in dark habitat. The other half, equal numbers
00:08:40.06 of light and dark, in light habitat. And then come back, let's say a few
00:08:43.21 months later, and see who survived. And I'd have the expectation
00:08:46.22 that mice that are lighter would survive better in light habitat,
00:08:49.11 and those that are darker in dark habitat. Now for a number of reasons,
00:08:53.03 that experiment is quite hard to do. So instead, what we
00:08:56.16 did is what I'd argue is the next best experiment. And in some ways,
00:08:59.17 it may be even better. So here's the experiment that we actually
00:09:02.22 did. Instead of using live mice, we made mice. So here's
00:09:07.05 a picture of my postdoc, Sacha Vignieri, who along with
00:09:10.05 an undergraduate from Harvard, Joanna Larson, made
00:09:13.09 hundreds of plasticine mice. Half of them were painted
00:09:17.13 dark to mimic the mainland mice, and half of them were painted light
00:09:20.18 to mimic the beach mice. Now this experiment in some
00:09:24.28 ways, as I mentioned may even be better than using live
00:09:27.20 mice, because here the only difference in these mice is
00:09:31.04 their color. So they're made from the same mold, they all look the same,
00:09:34.09 they all smell the same. Whereas with live mice, the beach mice
00:09:37.09 and mainland mice may differ in let's say smell, in escape
00:09:39.28 behavior, in activity patterns. So here, the experiment completely
00:09:44.08 focuses on the difference in color and not correlated traits.
00:09:47.05 But the downside of this experiment is would it actually
00:09:50.18 work? Could we actually fool predators into attacking these
00:09:54.00 plasticine models of mice and not live mice?
00:09:56.29 Well, I wouldn't be telling you about this experiment if it didn't actually
00:09:59.26 work. So what Sacha did was she released equal numbers
00:10:03.22 of these light and dark mice in both light and dark habitats, where
00:10:06.20 live mice of the species actually occurred. And then counted
00:10:10.29 predation events. So here's a predation event. So what you're
00:10:13.29 looking at here is a dark mouse that was put out on light
00:10:16.16 soil. And you may notice that it's missing part of its
00:10:20.03 left ear, and it's got a big chunk taken out of its back.
00:10:23.05 And this is a classic predation event, and in fact, not only
00:10:26.26 can we tell it's been attacked, but we can actually say
00:10:30.08 something about who's doing the attacking. Because these marks
00:10:33.02 are consistent with an avian predator. So here's the results of
00:10:38.10 the larger experiment that Sacha did, where she was counting
00:10:41.14 the number of predation events in different habitat types.
00:10:44.23 So, what you're looking at here are the results of this experiment.
00:10:50.08 So let's first focus on this far panel at the light habitat.
00:10:54.17 What you can see is that there's cryptic and what we'll refer
00:10:57.11 to as non-cryptic mice. And these bars indicate the relative level of
00:11:03.01 predation in both of these two types of mice in this particular habitat.
00:11:06.28 And what you can immediately see is the level of predation here in
00:11:10.19 these cryptic mice is much lower, in fact, it's about half
00:11:14.00 of non-cryptic mice. So what this means is that both mice
00:11:17.22 were attacked by predators, but the mismatched mice were attacked
00:11:21.15 about twice as often. Now when we look at the dark habitat
00:11:24.28 we see a very similar pattern, but in reverse. Here we can
00:11:28.17 see the dark mice survived much better, and while still attacked,
00:11:33.22 they were attacked about half as often as the mismatched mice.
00:11:36.24 So what this first thing tells us is that color seems to matter.
00:11:40.10 And in fact it matters a lot. We can take these numbers and sort
00:11:44.16 of translate that into a selection intensity. I'm not going to go
00:11:48.12 into details about this, but let me just say that color matters
00:11:51.17 a great deal for these mice. And in fact, mice that match their
00:11:55.21 habitat have about a 50% increased chance of survival
00:11:59.21 compared to those that are mismatched. And the final thing
00:12:02.27 I want to say, as I mentioned, we can tell in some cases
00:12:05.08 who's doing the predating. And about half the attacks
00:12:09.16 were due to avian predators, and about half of the attacks
00:12:12.14 were due to mammalian predators like coyotes and foxes.
00:12:16.03 So together, what this experiment is telling us is that
00:12:20.06 the differences between color variation are tightly linked
00:12:22.17 to the environment and that it's natural selection that
00:12:26.01 is playing a role in driving these color differences. So now that
00:12:30.18 we've implicated a role for natural selection, the next thing I
00:12:34.00 want to do is to take you from the field into the lab,
00:12:36.15 where I'll tell you about how we're going about identifying
00:12:39.15 the genes that are responsible for these differences in adaptive color
00:12:42.12 variation. So the first thing I want to do is give you a general sense
00:12:47.23 or an overview of the approach that we're taking. And I don't
00:12:50.22 want you to get caught up in the details, but more appreciate
00:12:53.01 this general approach. So what we're able to do is
00:12:57.05 take these mice from the field and bring them into the lab, so
00:13:00.13 we have both dark mice and light mice. And they have
00:13:03.27 differences in their genomes, in their chromosomes, so
00:13:06.24 I'm going to illustrate this by the dark mice having dark chromosomes
00:13:09.27 and the light mouse having light chromosomes. And what we can
00:13:12.29 do is take a dark mouse and a light mouse, one male and one
00:13:15.05 female. Put them in a cage together and they'll actually
00:13:17.11 reproduce. And they'll produce what we refer to as hybrids.
00:13:21.04 Then we take those hybrid individuals, and we breed them
00:13:24.20 together. And then what happens is in this next generation
00:13:27.16 is their genomes get shuffled. So some individuals are going to have
00:13:31.16 different parts of their chromosomes that come from the light parent,
00:13:34.08 and some from the dark parent. So we're effectively shuffling
00:13:38.06 up their genomes. Now in this population, this second generation of hybrids,
00:13:42.13 what we do in all those individuals is we measure their coat
00:13:45.16 color pattern, and then using genetics or molecular biology,
00:13:49.16 we're able to sort of characterize their chromosomes and determine what regions
00:13:53.08 come from each of the parents. And then what we do is
00:13:56.16 -- I'll just simplify and say we do a nice statistical analysis
00:13:59.20 and ask, are there regions of the genome that seem to be
00:14:03.05 correlated with different aspects of different color variation?
00:14:06.20 So for example, in these chromosomes here, do all these mice
00:14:10.08 that have the light allele from this parent in this region
00:14:15.04 of the chromosome, if all those mice have, let's say, light
00:14:18.13 tails. That suggests that a gene controlling tail color
00:14:21.20 may reside in this part of the chromosome. And this is what we
00:14:25.11 refer to as a QTL analysis, or quantitative trait locus analysis.
00:14:29.03 But it's really just this simple statistical association.
00:14:32.10 And then what we do with this statistical association is that
00:14:36.06 we take that region of the chromosome, and we lookfor its homologous region, the same region in the mouse
00:14:43.01 genome, which we have the complete genome sequence.
00:14:45.12 We know where all the genes are. And we look for
00:14:48.02 candidate genes, remember that list of candidate genes
00:14:51.07 that I showed you earlier in the talk, and ask do any of those
00:14:54.04 candidate genes fall within this particular chromosome region.
00:14:57.16 With the ultimate goal of finding a mutation in that gene
00:15:01.04 whether it's in the protein structure of that gene or
00:15:04.10 maybe a mutation that controls the regulation of this gene,
00:15:07.21 that we can then link to the color differences between the parents.
00:15:10.24 So now that I've given you this overview, next what I want to do is walk
00:15:14.02 you through each of the pieces. So as I mentioned the first
00:15:17.02 thing we do is this cross. So we brought mice in from the wild,
00:15:21.15 we brought in a mainland species and one of these beach
00:15:24.09 mouse subspecies. And here they are, shown again in their
00:15:26.28 flattened form. You can see a dark parent, and over here
00:15:29.13 the light parent. And here is that F1 hybrid shown here. And you can
00:15:34.16 already see this F1 hybrid has traits from both of the parents.
00:15:38.26 So for example, it doesn't have a tail stripe like the light parent
00:15:41.29 but it has a fully pigmented face like the dark parent.
00:15:45.01 That suggests both dominant and recessive alleles
00:15:49.01 contribute to this light color adaptive beach mouse
00:15:52.23 phenotype. Then as I mentioned, we take these F1 hybrids and we breed
00:15:56.06 them together, and that's when we get these F2s.
00:15:58.23 And this is where we've now with recombination shuffled
00:16:02.00 up their genomes. So, for example, presumably this mouse
00:16:05.22 has more pigment alleles from the light parent, and the mouse over here
00:16:10.08 has more pigment alleles from the dark parent. But what you can
00:16:13.05 see is that there's a continuum of variation. And what this
00:16:16.11 immediately tells us that this color difference we observe
00:16:19.11 between beach mice and mainland mice is not controlled by
00:16:22.06 a single gene, but in fact is controlled by a handful of genes.
00:16:26.10 And the reason I say a handful and not hundreds is because
00:16:29.04 you can see in this population we get mice that look like
00:16:31.15 the dark parent, and we get mice that look like the light parent.
00:16:34.16 And this suggests there are not hundreds of genes, because it would
00:16:37.26 be a very small chance that we would get all the light
00:16:40.11 -- all hundred of those light alleles in one individual.
00:16:43.06 But instead, probably just a handful. And from this variation,
00:16:46.27 we estimate there are about 3-5 genes. But I'll tell you more about
00:16:50.05 those genes in just a minute. So as I mentioned, we then take
00:16:53.19 the color variation in these mice and we measure them in
00:16:57.01 all these individuals. And then we also genotype them to
00:17:00.08 figure out what regions of the genome come from the light and dark parent.
00:17:03.06 We did this using molecular techniques. And here's the results
00:17:08.10 of that. So what this is is each one of those lines shown
00:17:11.29 here represents a chromosome. And each one of these markers is
00:17:15.14 a difference between the light and the dark parent.
00:17:17.12 So in each one of these markers we can tell whether a particular
00:17:20.08 individual has that region of the genome comes from the dark
00:17:23.05 parent or the light parent. Then what we do is the statistical
00:17:27.05 analysis. And what we found was, there are three regions
00:17:30.22 of the genome, which I've highlighted here, that seem to
00:17:33.27 control color. That is there's genes in these regions of
00:17:36.15 the genome that control different aspects of color patterning.
00:17:39.25 And lucky for us, in each one of these regions, there's one
00:17:44.17 of these candidate genes that I mentioned earlier.
00:17:47.00 Oh, I should also mention that the differences in the size
00:17:50.12 of the arrows reflects the amount of variation that a particular
00:17:53.14 locus explains. So the Agouti locus way over here, explains
00:17:58.12 a larger proportion of variation compared to Corin, which explains
00:18:02.05 the smallest amount. So what this is telling us is that there's
00:18:06.06 3 regions of the genome, and each one of those contains
00:18:08.29 what I'll refer to as a candidate gene. So next what I'd like
00:18:12.13 to do is just tell you about what of these genes. And then I'll
00:18:15.22 summarize and tell you about all three of them at the end.
00:18:17.28 So the gene I'm going to focus on is this gene up here,
00:18:21.07 Mc1r, or the melanocortin 1 receptor. And one of the
00:18:24.26 reasons we focused on this gene is because we actually
00:18:27.13 know a lot about the structure and the function of this gene.
00:18:30.03 So, the melanocortin receptor is a classic g-protein coupled
00:18:35.24 receptor. That is, it's found in the membrane, and it's got
00:18:39.18 extracellular and intracellular regions. Each one of these
00:18:43.22 little circles represents a different amino acid, and what
00:18:46.06 I've done is I've color coded those amino acids that we know
00:18:49.23 when you change that amino acid, it has an effect on
00:18:52.29 color, and on particular species. And those that make
00:18:56.20 -- when you make that change, an individual darker, it's shown
00:18:59.24 in black and those lighter is shown in gray. So you can see
00:19:03.12 summarized over a number of different studies, that
00:19:07.13 there are multiple mutations in this receptor that can affect
00:19:11.06 color. And it can either make an individual lighter or darker.
00:19:14.20 So the first thing to note is that there are many different
00:19:17.17 mutations. The second thing to note is that they're found throughout
00:19:20.28 the receptor. And the third thing to note is that even a single
00:19:23.27 amino acid mutation can have an effect on color. So one change
00:19:27.26 can have a big effect on phenotype. Now, the first thing we did is
00:19:32.29 we sequenced this gene in both the mainland and the beach
00:19:37.24 form, and asked are there mutational differences between
00:19:40.15 the two? And in fact, we found one and it's highlighted
00:19:44.03 here in red. And the mutation is a single nucleotide change that
00:19:48.03 caused the change in amino acid, whereas the mainland species had
00:19:51.20 an arginine at position 65, beach mice had a cysteine.
00:19:56.02 And this is a charged changing mutation, so it actually
00:19:58.21 changed the charge of that amino acid so that it's more likely
00:20:01.06 to have an effect on the structure and function of melanocortin
00:20:04.14 1 receptor. Now the unfortunate thing, though, was that it didn't
00:20:09.03 overlap with any of the other mutations that had been previously
00:20:11.07 characterized in other species. But that's okay because we can do an experiment
00:20:14.18 to test whether this mutational change had an effect on the way
00:20:18.03 that this receptor functions. So first thing I want to do is tell you a little bit
00:20:21.25 about what this receptor does. And then I'll tell you about our
00:20:24.25 experiment. So what I'm showing you here is a melanocyte.
00:20:28.19 Now a melanocyte is a pigmentation producing cell.
00:20:31.07 And in mammals, we produce two types of pigment,
00:20:34.03 a dark brown to black eumelanin, and a yellow to blonde
00:20:37.19 pheomelanin. So you can look at your own hair and
00:20:39.12 determine whether you have eumelanin or you have pheomelanin.
00:20:42.25 Now, this melanocyte has the ability to produce both types
00:20:47.21 of pigments. But which pigment it produces at any one time
00:20:50.14 is largely controlled by the melanocortin-1 receptor.
00:20:54.05 Which essentially acts like a switch. When it's turned on,
00:20:57.11 that is when let's say alpha-MSH, which activates Mc1r,
00:21:02.03 is around, Mc1r turns on and it signals by increasing intracellular
00:21:06.11 cyclic AMP levels, and you get the production of dark pigment.
00:21:10.00 By contrast, when Mc1r is turned off, then you get the production
00:21:15.15 of less cyclic AMP intracellularly, and the result is light
00:21:19.21 pigment. So Mc1r is very much a switch that determines which pigment is
00:21:24.27 produced. Now this leads to a nice prediction. So in beach mice we have
00:21:29.03 a mutation in Mc1r that we think leads to light coloration.
00:21:32.14 So our prediction is this, is that mutation of arginine to cysteine
00:21:36.18 change at position 65 will reduce receptor activity, which will result
00:21:41.13 in lighter pigmentation. So we can test this doing an experiment.
00:21:45.02 And so what we did is we took both the light allele and the dark
00:21:49.03 allele, that remember, differs by one nucleotide change,
00:21:51.26 we cloned it into an expression vector, we put it into
00:21:55.03 cells. And then we added alpha-MSH in increasing amounts.
00:22:01.14 To activate Mc1r, and then as a proxy for activity, we measured
00:22:05.13 cyclic AMP. So here are the results of this experiment.
00:22:09.17 So here what we did is you can see we added increasing
00:22:14.16 amounts of alpha-MSH, which turned on Mc1r, and you can
00:22:18.21 see that its signaling higher and higher cyclic AMP
00:22:21.22 until it sort of plateaus out. And this is a normal sigmoidal response curve
00:22:26.09 that we see in the mainland mouse. Now when we did the same
00:22:30.02 experiment with the beach mouse allele that differs by again
00:22:32.22 that one nucleotide change, what you can see is there's a dramatically different
00:22:36.14 pattern of activity. And that is no matter how much alpha-MSH
00:22:40.28 we added, there's still a relatively low level of receptor activity.
00:22:44.21 So what this suggests is that one nucleotide change changes the
00:22:48.26 function of the receptor, and it's in the direction we expect.
00:22:53.04 That it has lower activity, which is consistent with producing
00:22:55.21 lighter pigmentation. So at this point, it's worthwhile stepping
00:22:59.07 back and sort of thinking about what I've just showed you. So here I've
00:23:02.22 showed you a single nucleotide change affects the activity of the receptor,
00:23:07.04 we know this receptor affects color variation, and we know
00:23:11.08 that color variation affects survival in the wild. So what we
00:23:14.13 have done here is made a link between a single nucleotide
00:23:17.14 change and fitness or survival in the wild. But I don't want
00:23:22.24 to leave you with the impression that this is the whole story, because
00:23:26.28 as I mentioned, color difference is controlled not by a single
00:23:29.03 gene, but by multiple genes. And so the two other genes,
00:23:33.23 if you are paying attention you might have noticed, actually
00:23:37.02 interact with Mc1r. So Agouti, for example, represses Mc1r
00:23:42.02 activity. And what we see in beach mice, is that higher
00:23:45.26 levels of Agouti expression are associated with lighter pigmentation.
00:23:49.07 And so the allele in beach mice has increased expression
00:23:52.13 of Agouti, which leads to lower activity of Mc1r and light pigment.
00:23:57.02 Corin is the third gene, and while this gene is just newly
00:24:02.04 discovered in the last few years. We don't know its exact function
00:24:05.14 but we know it interacts with Agouti, and again, increased
00:24:09.05 expression of Corin is associated with this light pigmentation
00:24:11.26 in beach mice. So these genes are interacting together
00:24:15.11 to produce light pigmentation. So what we've done in this second part
00:24:20.28 is to tell you about the genes that affect coloration and a little
00:24:25.08 bit about how changes in those genes actually cause
00:24:28.09 these differences in color patterning. And now that we have a much
00:24:32.20 more complete picture of adaptation, here's where I think things can get
00:24:36.08 really fun. So I'm going to take you now back from the lab into the
00:24:39.16 wild. And we're going to talk about how these traits may have
00:24:42.05 evolved in natural populations of beach mice. So to do this, this
00:24:46.14 involves us actually going back to the field. So here's a picture
00:24:49.15 of me and my postdoc, Vera Domingues, when we're out
00:24:53.04 on the Atlantic coast catching mice. Here's a mouse
00:24:57.07 currently being weighed. So we hang it by its tail on a little
00:25:00.09 pesola. We take measurements including their weight,
00:25:03.15 the size of their ears and feet, et cetera. And what Vera's doing here
00:25:07.20 is measuring their coat color. And what's nice about this
00:25:11.23 work in the wild is we take these measurements, we measure their
00:25:14.21 coat color, we also give them a little ear tag, and we take a little
00:25:18.05 snippet of DNA from their tail, and we release them back
00:25:21.19 in the wild. So here we have a DNA sample from each of
00:25:24.12 these mice and we have a record of their coloration.
00:25:26.24 So next what I'd like to do is tell you about what we've learned
00:25:30.02 about natural populations of these mice and how these color
00:25:33.15 differences may have evolved. So just by way of reminder,
00:25:36.29 so far what I've done is I've focused on only one of these
00:25:40.22 populations, that is population number 3, the Santa Rosa
00:25:42.29 Island beach mouse. But next what I'd like to do is tell you about
00:25:46.02 variation among these subspecies. So the five subspecies
00:25:49.26 on the Gulf coast and the three subspecies on the Atlantic coast.
00:25:53.13 So, when we go out and catch these mice and record their
00:25:58.12 color differences. We find some very striking patterns, which I'm going to
00:26:02.19 show you in the next slide. So here what I'm showing you
00:26:05.18 are cartoons that represent the different subspecies of
00:26:10.12 beach mice. So each one of these cartoons shows you the typical
00:26:14.09 color of a beach mouse from each of these populations, compared
00:26:17.07 here to a mainland mouse. So the first thing you may notice
00:26:21.19 is that all the beach mice are much lighter in color
00:26:26.00 compared to the mainland mouse. But that each of these
00:26:28.07 subspecies differs in their color pattern, and in fact,
00:26:31.23 they're so distinct that if you went for let's say spring break
00:26:35.06 down to the Gulf coast of Florida and you brought me back a
00:26:38.13 beach mouse, I would say with about 95% certainty
00:26:42.03 just by looking at the color of the beach mouse, I could tell you
00:26:44.16 what subspecies it is. But, instead if you went to Florida and you
00:26:49.09 didn't tell me if you went to the Atlantic coast or the Gulf
00:26:51.28 coast, and you just brought me back a beach mouse, I'd probably
00:26:55.08 have a 50/50 chance of knowing what subspecies it was
00:26:58.17 and that's because the mice on the Atlantic coast are very similar
00:27:02.09 to mice on the Gulf coast. So let me highlight that here.
00:27:05.26 So for example, these two subspecies, even though they're
00:27:08.29 separated by over 300 kilometers, are very similar
00:27:12.18 in their overall color pattern. And in fact, I can't tell them
00:27:16.10 apart. Likewise, these mice are very similar. And these mice
00:27:20.26 are very similar. So what this suggests is that on the Atlantic
00:27:26.02 coast and Gulf coast, the mice have convergent color patterns.
00:27:31.08 And so what we wanted to do first was ask the question,
00:27:34.16 did these mice evolve these similar color differences independently?
00:27:39.14 And if so, did they use the same genes? So the first
00:27:44.02 thing I want to show you is a tree, or a topology that shows
00:27:48.24 you the relationships among these different subspecies.
00:27:52.00 So this is a simplified version of a tree that we generated
00:27:54.28 using molecular data, but it highlights the relationships
00:27:58.12 among these subspecies within peromyscus polionotus.
00:28:02.07 And what you can see is the Gulf coast beach mice, shown here,
00:28:06.08 all five of those subspecies cluster together. They're very
00:28:09.09 closely related. But they're actually not that closely related
00:28:12.16 to Atlantic coast beach mice, shown here. In other words,
00:28:17.11 it looks like light coloration has evolved independently
00:28:20.02 on two coasts. So the Gulf coast beach mice probably
00:28:23.07 arose from a dark colored ancestor sometime in the past,
00:28:26.22 that was probably from the Panhandle of Florida. Whereas,
00:28:29.23 the Atlantic coast beach mice independently evolved light
00:28:32.20 coloration, probably from an ancestor in Central Florida
00:28:35.26 that was dark in color. Okay, but here's the cool part,
00:28:40.04 now that we now at least in one of these Gulf coast subspecies
00:28:43.07 that the melanocortin-1 receptor is involved, we can
00:28:45.22 ask in these independently evolved light colored beach mice
00:28:48.22 on the Atlantic coast, is that same gene and same mutation
00:28:51.15 involved? So to do this, we returned back to this melanocortin-1 gene.
00:28:56.21 And we sequenced the DNA in the mice that we collected
00:28:59.19 on the Atlantic coast and asked, do we find that same
00:29:02.24 arginine to cysteine change at position 65? So we simply
00:29:07.28 genotyped that one particular site and asked, is it present in the
00:29:12.00 Atlantic coast? Now, despite the fact that mice from the Gulf
00:29:15.09 coast and the Atlantic coast are so similar in coat color,
00:29:18.06 let me just say that we never found that cysteine change in any of the
00:29:22.09 mice from the Atlantic coast. So what that tells us is that
00:29:26.01 the same mutation isn't involved in that convergently evolved
00:29:29.20 light coloration on the Atlantic coast. So you may be thinking,
00:29:32.13 well, it's not the same mutation, but maybe it's a new mutation.
00:29:36.09 Remember there's lots of mutations in Mc1r that can cause
00:29:39.08 color differences, I told you this earlier. So maybe a new mutation
00:29:42.12 in Mc1r is causing light coloration on the Atlantic coast. So
00:29:45.23 it may not be the same mutation, but it could be the same gene.
00:29:48.18 So we went back to the Atlantic coast mice and sequenced
00:29:50.20 the entire melanocortin-1 receptor, and asked are there any
00:29:54.08 new mutations that are correlated with color. Well, in fact,
00:29:57.08 we found four new mutations in the melanocortin-1 receptor.
00:30:01.02 But none of them were perfectly correlated with color, and
00:30:04.11 when we did those pharmacological assays like the ones
00:30:07.04 I showed you earlier, none of them had an effect on the
00:30:11.17 activity of Mc1r. So what this tells us is that it's not just
00:30:16.12 the same mutation, it's also not the same gene that's
00:30:19.11 responsible for the convergent evolution of light coloration in the
00:30:23.10 Atlantic coast mice. So this is the case for melanocortin-1 receptor,
00:30:26.25 but we're still checking these populations for changes in Agouti and Corin.
00:30:31.27 So what I've done today is I've told you a story about
00:30:37.00 how identifying not only the ultimate causes of phenotypic
00:30:41.12 variation, but also the genetic causes can tell us something about how
00:30:44.13 traits evolved in the wild. And this story revolved around a single
00:30:47.24 species, in which different mutations, at least in regards to
00:30:52.04 the melanocortin-1 receptor, are involved in generating
00:30:55.04 similar coat color patterns on two coasts of Florida.
00:30:57.25 But I want to end by telling you a story about convergent
00:31:01.14 evolution. Because sometimes the same genes and same
00:31:04.13 mutations are involved in similar phenotypes in very different
00:31:08.01 organisms. So, if you think about mammals, think about what
00:31:13.02 is the most different mammal from a mouse. Often the answer
00:31:17.03 I get is an elephant, and it's close. I'm going to tell you a story about
00:31:21.11 mammoths. So, mammoths were the subject of intense
00:31:26.17 study, especially about five years ago when there was a big
00:31:30.06 interest in sequencing ancient DNA. And mammoths were
00:31:33.28 a great target because they occurred in Siberia, and
00:31:38.05 their DNA was essentially frozen in permafrost about 14,000
00:31:43.03 years ago. So this is almost like keeping DNA in a giant freezer,
00:31:46.15 which is the best conditions possible. So about five years ago,
00:31:50.07 the goal was to sequence an entire gene from an extinct
00:31:53.25 organism. Now today of course, we're sequencing entire
00:31:57.03 genomes of extinct organisms, but just five years ago, we
00:32:01.00 wanted to sequence an entire gene from the nuclear genome.
00:32:04.29 So this is what a group in Germany, in Leipzig, headed by
00:32:10.12 Michael Hofreiter did. And the gene they chose to study
00:32:14.00 was the melanocortin-1 receptor, because it's a very
00:32:17.13 simple gene. Remember I told you, we know a lot about its
00:32:20.27 structure function, it's only about 1,000 base pairs in length,
00:32:23.05 and there's no introns. So it's very simple and it's a great starting
00:32:26.05 point. So they sequenced the melanocortin-1 receptor,
00:32:29.10 in DNA extracted from mammoths. And here's what they found.
00:32:35.14 They found a mutation, and what mutation was it?
00:32:39.01 Well, it was an arginine to cysteine change at the exact
00:32:41.19 same position that we found in beach mice. Now of course
00:32:45.15 the DNA it was extracted from was bone, so they didn't know
00:32:48.18 the phenotype or the coat color of mammoths, but based on our
00:32:52.06 work in beach mice, what this suggests is that mammoths
00:32:55.01 like beach mice, may have been polymorphic in color. Now the
00:32:59.07 question of why they were polymorphic in color, we don't know the
00:33:01.26 answer to. This could be due to survival differences, other
00:33:06.25 suggested that it's due to sexual selection. So when this
00:33:10.07 work was published, the press line was that blonde mammoths have
00:33:14.15 more fun. But I'm not going to give you any explanation, I'll leave that
00:33:19.08 up to your imagination. So in this case, we have very
00:33:22.21 divergent organisms, mice and mammoths that use
00:33:26.03 not only the same gene, but the same mutation.
00:33:29.12 And in fact, as I alluded to earlier, melanocortin-1 receptor
00:33:33.06 is involved in a number of different color variants.
00:33:36.05 And a number of organisms that you may be familiar
00:33:38.24 with. So in this case, it was the same mutation, but we know
00:33:42.25 that other changes in the melanocortin receptor can also
00:33:46.21 cause differences in color through different mutations. So I just wanted to
00:33:51.02 give you some examples. So some work that I've been doing with a colleague
00:33:54.12 at UC Berkeley, Erica Rosenblum, shows that changes in the
00:33:57.26 melanocortin-1 receptor is responsible for the production of these
00:34:00.22 very adorable lizards that differ in color, when they occur
00:34:05.14 in White Sands, New Mexico. So you can guess which
00:34:09.02 lizard occurs on white sands and which one occurs off white sands.
00:34:13.06 Color differences are also involved in animals you may see
00:34:17.09 every day, including cows. Also, many of you may know
00:34:22.12 of or even have a labrador dog. Well, they come in
00:34:26.21 generally two colors, there's the black labs and blonde labs,
00:34:30.12 again this is caused by a change in the melanocortin-1 receptor. And much more recently, again work out of Germany,
00:34:36.23 this time Svante Paabo's group, has shown that color
00:34:41.06 changes in the melanocortin receptor, in addition to being
00:34:45.20 responsible for human color differences, may also be
00:34:48.29 responsible for color differences or hair differences in neanderthals.
00:34:53.03 So they showed that changes in the melanocortin-1 receptor
00:34:56.02 or variants in the melanocortin-1 receptor were found in
00:34:59.24 neanderthals, suggesting even back in those days,
00:35:05.03 there could have been redheads as well. So what I hope to have done
00:35:09.07 today is to suggest to you that by making the link between
00:35:13.23 environment, phenotype -- in this case a morphological trait,
00:35:17.22 and genotype, we're able to say something about
00:35:21.05 how organisms evolve in the wild. The work that I presented
00:35:25.12 today was done by a large number of people. These are some
00:35:30.22 of the people in my lab group that contributed to the work,
00:35:34.05 shown here looking their mousiest. And in particular,
00:35:38.00 the work I talked about today was done by folks like
00:35:41.21 Cynthia Steiner, Marie Manceau, Vera Domingues, Sacha
00:35:44.23 Vignieri, Holger Rompler, and Lynne Mullen, as well as
00:35:47.29 an undergraduate, Joanna Larson. And we were funded by
00:35:52.03 a number of sources shown here, as well. And with that,
00:35:55.10 thank you for your attention. I hope you enjoyed this segment,
00:35:58.03 and you'll stick around for segment three. Thank you very much.
Related Resources
- Neil Shubin: Finding Tiktaalik, the Fossil Link Between Fish and Land Animals
- Sarah Tishkoff: African Genomics: Human Evolution and Migration
- Hopi Hoekstra: The genetic basis of Evolutionary Change in Morphology and Behavior
Speaker Bio
Hopi Hoekstra

After a short stint studying political science in college, Hopi Hoekstra switched her focus to biology. She received her B.A. in Integrative Biology from UC Berkeley, and her Ph.D. in Zoology from the University of Washington. She completed postdoctoral research at the University of Arizona, and, in 2003, she joined the faculty at UC San… Continue Reading
Neil Shubin
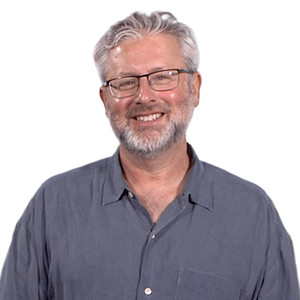
Dr. Neil Shubin is a Professor in the Department of Organismal Biology and Anatomy and the Committee on Evolutionary Biology at the University of Chicago. Shubin’s research focuses on understanding the evolutionary origins of new anatomical features such as limbs. Shubin is well known for his discovery of Tiktaalik roseae,the 375 million year old fossil… Continue Reading
Sarah Tishkoff
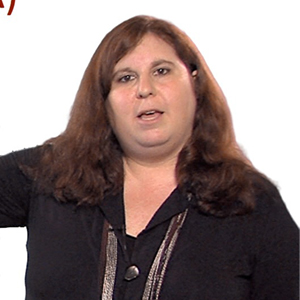
Sarah Tishkoff studied anthropology and genetics as an undergraduate at the University of California, Berkeley. She received her PhD in genetics from Yale University and was a post-doctoral fellow at Pennsylvania State University. From 2000-2007, she was a faculty member in the Department of Biology at the University of Maryland. Currently, Dr. Tishkoff is the… Continue Reading
Leave a Reply